Subsystems
Subsystems | Detection Type | Path | Comment |
---|---|---|---|
SiDSSD - XSiS | implant & charged particle | new | Good resolutoin for proton and α spectroscopy |
XScint - XSiS | implant & charged particle | new | Fast segmented scintillator |
GeDSSD | implant & charged particle | exists | High resolution detection of X-rays and conversion electrons |
TPC | implant & charged particle | exists | Correlated charged-particle detection |
HPGe Clovers - DEGA | γ rays | new | High-resolution, large-volumne (4 crystals x 7 cm x 8 cm), and tapered |
BGO Shields - DEGA | γ rays | new | Recessed Compton-suppression coverage for high efficiency configuration |
HPGe PCs - DEGA | γ rays | new | Large face and very high resolution at low and medium energies |
LaBr3 | γ rays | upgrade | Fast timing and good resolution |
NaI(Tl) - MTAS / SUN | γ rays / neutrons | upgrade | Segmented total absorption |
3Hen | neutrons | upgrade | High segmented and efficiency neutron counter |
n TOF - NEXT | neutrons | new | Good resolution, large solid-angle and efficiency, granular, discrete neutron spectrometer (pairs with XScint for TOF) |
XSiS (SiDSSD + XScint)
Silicon double-sided strip detectors (SiDSSDs) have traditionally been the workhorse for experiments with fragmentation beams because of their good energy resolution for charged particles (20-50 keV FWHM), adequate timing characteristics, and compatibility with most γ-ray detector arrays. SiDSSDs will be particularly well suited for experiments targeting isotopes on the proton-rich side of stability because the β-delayed charged particles can be studied with these same detectors. The stopping power of silicon is low, necessitating several 1-mm thick layers to stop the heavy ions of interest and the electrical segmentation pitch must be 0.5 to 1 mm to handle the implantation rates and subsequent decays. In addition, a large dynamic range is required to handle both high-energy implants and low-energy decays in order to maintain good energy resolution for each case. However, for the n-rich side of stability, a fast position-sensitive inorganic scintillator (XScint) is required for neutron TOF measurements with NEXT. In fact, there are merits to both types of detectors for both sides of stability. A preliminary design of the XScint-SiDSSD hybrid array (XSiS) layout is shown on the right. This detector is expected to achieve an implant-β efficiency of up to 75%, which is nearly a factor of 2 larger than the previous generation implant detector, NSCL-BCS.
The XSiS detector design could also enable parallel measurements with a downstream total absorption/counting spectrometer by allowing an adjustable amount of beam through, e.g., 10%. This would be a completely new capability for the FDS that is not provided in a previous generation equivalent of this detector.
GeDSSD & — Germanium Double-Sided Strip Detector
These implant detectors have become available within the last 5 years and have been shown to be a very efficient tool for detecting β particles and conversion electrons. The implants are stopped in a single 1-cm thick detector and βs are easy to identify. This detector is ideal for the most exotic isotopes with low production rates and for measuring conversion electrons. This system will be a workhorse for experiments targeting shape coexistence and isotopes on the neutron-rich side of stability.
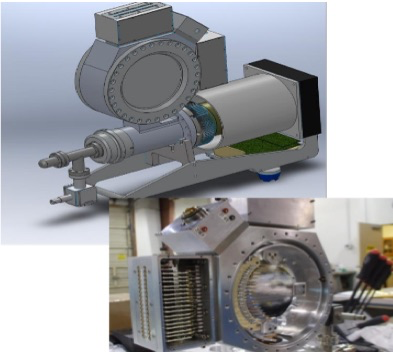
TPC — Time-Projection Chamber
Gas implantation detectors can be used to directly image the charged-particle decay tracks. They stop the ion in the gas where the subsequent decay is observed. Betas are not observed in the gas, making the identification of direct and β-delayed (multi-) proton and α emission simple. This is achieved by using high segmentation (TPC) for high count rate applications or light scintillation (optical TPC) for more exotic decays. The planned TPCs will be designed to fit inside a γ-ray detector array, DEGA.
DEGA — DEcay Germanium Array
An efficient, high-resolution, granular array of Compton-suppressed HPGe detectors will be an essential component of the FDS. The DEcay Germanium Array (DEGA) will be used primarily to detect β- and isomer-delayed γ-ray transitions with high energy resolution in exotic nuclei produced at FRIB. It will be used in conjunction with other detectors such as implantation detectors, neutron detectors, and scintillator arrays. Compton suppression will not only improve the peak-to-total (P/T) of the γ-ray spectrum, but it will also suppress correlated background in γ-γ coincidence measurements that arise from Compton cross scattering.
Large-volume and tapered (22.5o) clover detectors, which house 4 crystals (7 cm diameter x 8 cm length each) per cryostat and can be closely packed, are a good compromise between cost and performance. They also have excellent energy resolution (FWHM ≤ 2.5 keV at 1.33 MeV) and “back catchers” for extra coverage by recessed BGO Compton- suppression shields, which will provide excellent peak-to-total (≥50% at 1 MeV). Simulations indicate that the larger crystals, as compared to the old 5×8-cm style clovers, will alone improve the P/T by ~20% at 1 MeV. The addition of back catchers will further improve the P/T by as much as 10%; this becomes more substantial for higher energies. New recessed shields are required for high-efficiency configurations of any clover type. In order to enhance the sensitivity of such an array at low energies, thin-windowed, p-type point-contact detectors (PCs) are required (~9 cm diameter); a newly implemented technology that provides outstanding energy resolution (FWHM ≤ 0.6 keV at 100 keV) and efficiency at low energy. Sensitivity in this energy range is important for studies of isomers (e.g., seniority isomers), X-rays, and decays in heavy- and odd-mass isotopes. Simulations for the entire array (72 crystals) indicate photopeak efficiencies of up to 23(2)% at 1 MeV and 65(7)% at 100 keV; there are “push-in” configurations that can take the array to even higher efficiencies but at the expense of granularity. These efficiencies are nearly double that achievable with the old 5×8-cm style clovers. The symmetry and granularity of the spherical HPGe array (72 crystals) is ideal for angular correlations and medium-to-large multiplicities. It is also ideal for matching to 2π configurations of other detector arrays; e.g., the neutron TOF array NEXT.
LaBr3 — Lanthanum Bromide array
The LaBr3 detector array represents an appealing combination of many γ-ray detection characteristics: energy resolution (3% at 1.3 MeV), stackable for high efficiency at high energy when used with silicon photomultipliers (SiPM), and fast timing (sub-nanosecond timing resolution). This array will be compatible with all implantation detectors, NEXT, and DEGA.
MTAS — Modular Total Absorption Spectrometer
The existing NaI(Tl) Modular Total Absorption Spectrometer (MTAS) was designed for and successfully used with low emittance ISOL beams. This 4π detector array contains 1 ton of scintillator and has an efficiency greater than 70% for collecting the full energy of 4-MeV γ rays. It is a well-characterized detector array that is used to determine the β-decay feeding to excited states of the daughter nucleus. A new central module and plug detector, modified to accommodate the extended size of fragmentation beams, will enhance performance for low-energy γ rays, improve the γ-γ coincidence capability and increase detection efficiency by closing off one of the two holes in the array. High β efficiency will improve MTAS sensitivity requiring compatibility with the GeDSSD, and/or YAP implantation systems.
SUN — SUmming NaI(Tl)
The SUmming NaI (SUN) detector at MSU is a γ-ray calorimeter with 98% angular coverage. It is a compact cylindrical configuration of NaI(Tl) crystals, 16 inches in height, and 16 inches in diameter, with a 1.8-inch borehole along its axis. SUN is segments in 8 optically isolated segments, housed in two semi-cylindrical housings, to minimize the amount of inactive material. SUN’s segmentation offers high sensitivity to individual γ rays, while its high efficiency provides sensitivity to the energy of the populated level. In the future, modifications will be made to accommodate larger auxiliary detectors at the center of SUN, and to also combine its high efficiency with high resolution capabilities. For this reason, we plan to pull the two halves of SUN further apart and fill the gaps with a combination of NaI and existing HPGe detector. See SUN Homepage.
3Hen — Helium-3 neutron array
The established neutron counting detector array 3Hen will be combined with other existing 3He detectors and augmented with new counters to exceed the efficiency of the BRIKEN system currently being operated at RIKEN. These detectors are encased in high-density polypropylene (HDPE) to thermalize the neutrons so they can react with the 3He. Gaps are present in the HDPE in order to place γ-ray detectors enabling neutron-γ correlations to provide definite isotopic identification as well as γ-γ coincidences. High β efficiency will improve sensitivity requiring compatibility with the GeDSSD and/or YAP implantation systems. Gamma-ray detection requires compatibility with Ge and/or LaBr3 detectors.
NEXT — NEutron (Xn) Tracking array
The next-generation neutron spectroscopy array will comprise layers of fast plastic scintillator detectors arranged in a hemispherical configuration and read out by position-sensitive light sensors. The NEutron (Xn) Tracking array (NEXT) will achieve a dramatic gain in neutron energy resolution over the energy range of 0.1-10 MeV as compared to the previous generation, e.g., VANDLE. This will be possible by improving both the timing resolution and the neutron interaction localization through the use of thinner, 0.5-cm thick, layers of fast plastic instead of the thicker 3-cm VANDLE bars. In addition, new generation materials enable neutron-γ discrimination for background suppression. The hemisphere will be located opposite to a γ-ray detector array. Each neutron detector module will be approximately 10″ x 3″ x 3″. The array can be operated in multiple time-of-flight configurations between 50 cm and 100 cm depending on the goal of experiment. This modularity will enable high-precision neutron energy distribution using long (100 cm) TOF base or detailed studies of multi-neutron emission in a more compact geometry (50 cm). At 50- and 100-cm TOF, the energy resolution at 1 MeV is expected to be 66 and 33 keV, respectively, which is a factor of 3 and 6 better than VANDLE. The 2π and 4π efficiencies of NEXT at 1 MeV are expected to be 29 and 57%, respectively, which are factors of 3 and 6 better than VANDLE.
NEXT will require compatibility with fast implantation systems. Addition of 6Li glass detectors or other new-generation materials will improve low energy (< 100 keV) neutron detection. Gamma-ray detectors are essential components of the new neutron array for complete measurement of decay cascades. Therefore, this array will nominally be operated in a hybrid configuration, where part of the solid angle is covered by high-efficiency γ-ray detectors, e.g., DEGA.